Biological Physics Comes of Age
Once an awkward confrontation between disciplines, biological physics is having its moment — and showing that life is not just a mess.
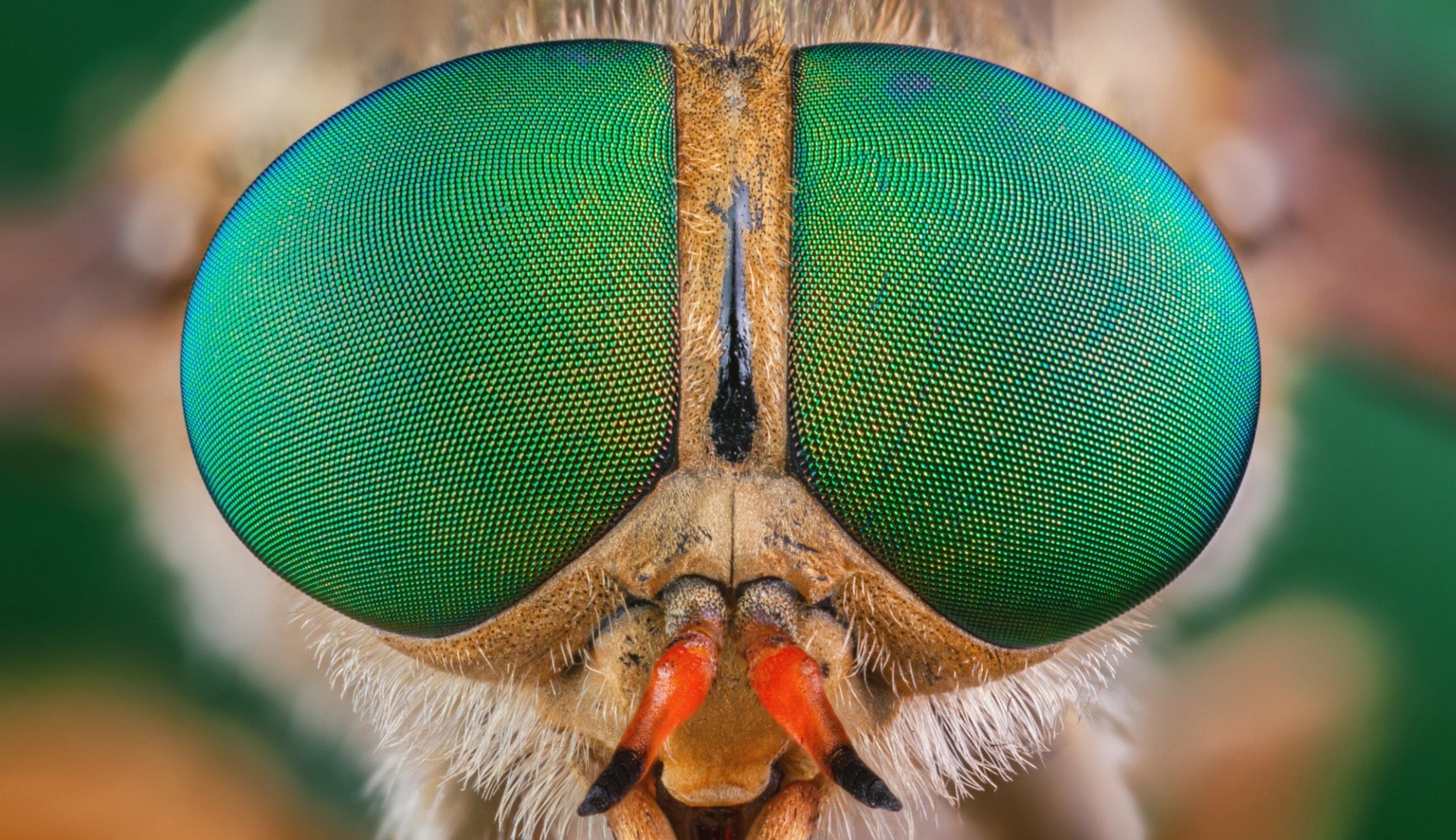
Physics and biology were not always separate disciplines. In the 18th century, controversy about “animal electricity” proved foundational for the understanding of electricity more generally. In the 19th century, explorations of vision and hearing intermingled with the emerging understanding of optics and acoustics. In the 20th century, the chasm between physics and biology grew wider, but there were spectacular bridges. Most famously, the work that launched our modern view of life — structures of DNA and proteins, theories of base pairing and the genetic code — was done primarily in physics departments.
The revolutionary successes of (re-)connecting physics with biology in the mid-20th century completely changed how we think about life, and even changed how biologists’ work is organized. What emerged first was called molecular biology, and over the course of a generation the ideas and methods of molecular biology — grounded in physics — touched almost every part of the biological sciences. In contrast, physics itself was left relatively untouched.
Although the interaction of physics and biology did not immediately change the trajectory of physics, a small stream of physicists continued to be fascinated by the phenomena of life. I began to be (dimly) aware of all this as a student in the late 1970s. For me, the things biologists talked about were interesting, but the way they talked about them was unsatisfying. Physics was the other way: the style of thinking was attractive, and the theories elegant and powerful, but I never had an original idea about problems in the field’s traditional core. It was clear that physicists were doing all sorts of interesting things connected to the living world, but these efforts didn’t cohere into a community and certainly not into a recognizable branch of physics. We went to meetings where (mostly) we would find biologists working on the same systems, but not physicists asking the same kinds of questions.
If we asked our biology professors, they would argue that a physicist’s search for simplicity and universality was in obvious conflict with the complexity and diversity of life. If we asked our physics professors, they might talk about a colleague who had "become a biologist," and perhaps warn that experiments in biology are messy. Discussions with both groups could easily be less polite than I have rendered them here. While physicists and biologists didn’t agree on much, they did seem to agree that it was a waste of time to try doing theoretical physics in the complex context of living systems.
Forty years later, the intellectual landscape has changed radically. At the APS March Meeting, we see physicists attacking an enormous variety of problems drawn from the living world. They wrestle openly with the complexity of biological systems while searching for general principles, doing what we easily recognize as physics. More and more young people are entering the field: The number of doctoral students who write their theses in biological physics today is essentially the same as the number who wrote theses in particle physics a generation ago. Our community is no longer trapped at the interface between disciplines, and biological physics has emerged as a branch of physics. These developments are celebrated in the recent report from the National Academy of Sciences, Physics of Life — the first survey of the field as part of the broader decadal survey of physics.*
As a community, we have made progress not by ignoring the complexity of life’s mechanisms, but by taming it, revealing underlying physics problems. An important part of what drove this progress was the interaction between theory and experiment, as in other areas of physics. Theory suggested new experiments and new ways of looking at data, and new experimental techniques made once-abstract theoretical questions concrete and urgent. We have examples from the mechanics of DNA and folding/unfolding of proteins, from the control of gene expression and the development of embryos, from the mechanics of single cells and flow of tissues, from collective behaviors in bacterial communities and flocks of birds, from networks of neurons and animal behavior, from immunology and evolution, and more.
In this spirit, my colleagues and I have studied intact biological systems whose functional behaviors are reproducible in the second decimal place, and we think this precision is key to theoretical understanding. We may be wrong about the theory, but I hope these (and many other) experiments will banish forever the physicists’ old suspicion that life is just a mess.
The focus on specific systems means that we now have rather solid understanding of the physics that underlies many particular phenomena of the living world. This is wonderful. At the same time, theoretical physics is not a collection of isolated models for particular phenomena. Has the price of concrete success been the loss of once grand ambitions? I hope not.
The mechanisms of life really are complicated, and theoretical physicists are intolerant of complication. At the risk of being cartoonish, I’ve tried to classify the paths that theorists in our community are taking to cut through this complexity.
One possibility is that we should give up on the quantitative connections between theory and experiment that we expect in physics more broadly. Instead, we should explore theories that remind us loosely of the real thing, and hope that we can capture general trends. This was an especially common view when experiments were messier than they are now. We continue to learn from these simpler models, but the experimental situation today is vastly different. Better data demand better theory.
A deeper possibility is that the search for simplification misses the point. Real biological systems are described by models with a huge number of parameters, and there is no escape. The interesting theoretical problems are how experimental data allow us to infer the parameters, or how prediction might be possible even if not all parameters are known. This might once have seemed like giving up, but the revolution in machine learning has taught us that “over-parameterized” models can be powerful, even if we’re still searching for a compelling theory of how and why this works. Progress toward such a theory should change how we think about the physics of life.
If deep networks are a guide, perhaps living systems have so many ways of solving the problems essential for survival that making a predictive theory of the underlying mechanisms is hopeless. Instead, we should focus on how one generation of solutions is related to the next — the dynamics of evolution. Importantly, evolutionary dynamics can have regularities and perhaps universalities even though individual evolutionary trajectories are unique. Statistical physics has been crucial in uncovering these regularities, and in making connections to a new generation of quantitative experiments.
A different possibility draws from statistical and condensed matter physics. Successful models for macroscopic behaviors often are much simpler than the underlying microscopic dynamics, and the renormalization group (RG) gives us a framework for understanding how this is possible. Many biological physicists hope that some RG-like arguments could help us extract more universal functional behaviors from the complex microscopic mechanisms of life, but turning this into a concrete program remains challenging. A successful example is in the analysis of collective animal behaviors, such as flocking and swarming. Related ideas come from dynamical systems theory, where behaviors near bifurcations or decision boundaries take on universal forms.
The last approach rests on the fact that living organisms are not arbitrary collections of microscopic components. Rather, they have been selected by evolution to perform functions crucial to life: converting energy, sensing the environment, coordinating movements, and more. In many cases, real organisms get close to the physical limits on their performance, as with diffraction-limited imaging and photon counting in vision, molecule counting in bacterial chemotaxis, and the efficiency of neural coding. These observations suggest a variational principle, optimizing functional performance subject to physical constraints, thus determining the parameters of very complex mechanisms without fitting. There is a long tradition of taking this approach to coding and computation in the brain, and my colleagues and I are excited about progress in using this approach to understand genetic networks.
This classification is neither complete nor orthogonal, but I hope it gives some idea of what our community has been trying to do. Each approach has its advocates and detractors, as in any lively field.
The community’s experimental mastery over living systems, across scales from molecules to groups of organisms, continues to grow. One can count (almost) every messenger RNA molecule in a cell, labeled by the gene from which it was transcribed, and one can track every bird in a flock of thousands. If current trends continue, more and more of these experiments will be coupled to theory. For me, and for many of my theorist colleagues, the question is whether something unified will emerge from these efforts on specific systems — something that has the power and generality that we are used to in the rest of theoretical physics.
The emergence of biological physics is a multi-generational project. But while physics today is broader and deeper than ever before, our teaching has not kept pace. Conveying the excitement of diverse new opportunities while still transmitting the culture that unites us as physicists requires integrating new fields into the core curriculum.
The message of biological physics is that the beautiful phenomena of life connect to deep physical principles. Bacterial swimming and sensing are dominated by low Reynolds number mechanics and diffusion. Vision and photosynthesis illustrate how quantum mechanics can produce broad absorption bands rather than narrow spectral lines. DNA exemplifies the random polymer that appears in all statistical mechanics courses. These and other examples can show students that physics reaches far beyond the world of inclined planes, isolated atoms, and ideal gases, to life itself.
Beyond these now classical examples lies genuinely new physics, topics of current excitement in our field. Realizing the promise of biological physics will change how we think about life, how we think about physics, and how we think about ourselves.
*As with all Decadal Surveys of Physics, this is a “consensus report,” reflecting a large committee’s deliberations. I had the honor of chairing this committee, but I write here as an individual. I encourage you to read the report for a broader view.
William Bialek
William Bialek is the John Archibald Wheeler/Battelle Professor in Physics, and a member of the multidisciplinary Lewis-Sigler Institute for Integrative Genomics, at Princeton University.